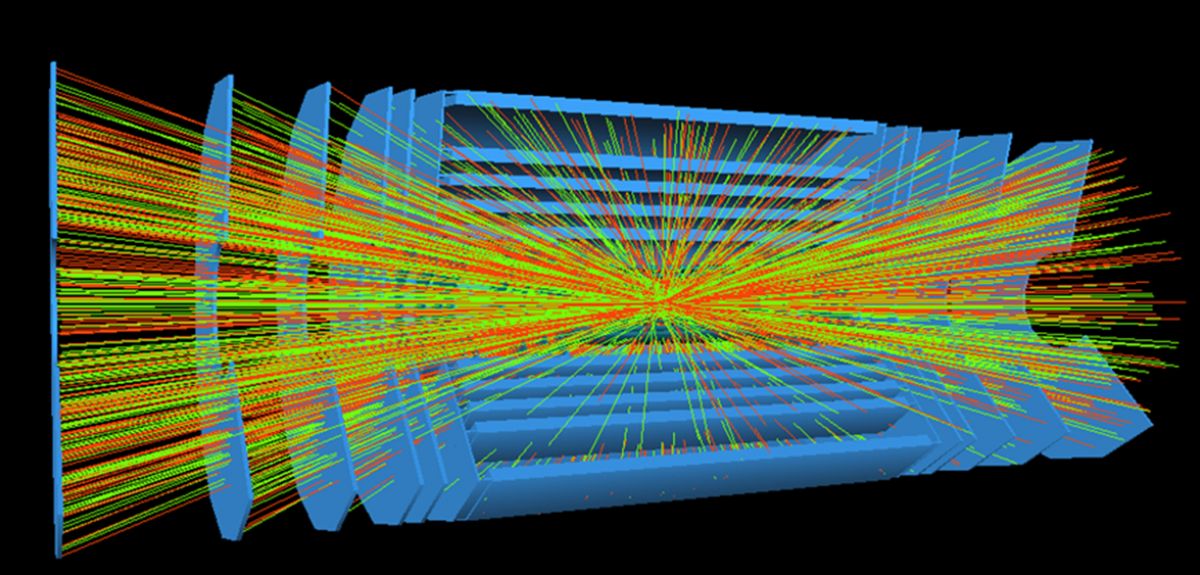
Image: CERN
Exploring the Higgs boson's dark side
In 2012 CERN's Large Hadron Collider (LHC) discovered the Higgs boson, the 'missing piece' in the jigsaw of particles predicted by the Standard Model.
Last month, after two years of preparation, the LHC began smashing its proton beams together at 13 Trillion electron Volts (TeV), close to double the energy achieved during its first run.
'We do not know what we will find next and that makes the new run even more exciting,' Daniela Bortoletto of Oxford University's Department of Physics, a member of the team running the LHC’s ATLAS experiment, tells me. 'We hope to finally find some cracks in the Standard Model as there are many questions about our universe that it does not answer.'
One of the big questions concerns dark matter, the invisible 'stuff' that astrophysicists estimate makes up over 80% of the mass of the Universe. As yet nobody has identified particles of dark matter although physicists think it could be the lightest supersymmetric (SUSY) particle.
'In the new run, because of the highest-ever energies available at the LHC, we might finally create dark matter in the laboratory,' says Daniela. 'If dark matter is the lightest SUSY particle than we might discover many other SUSY particles, since SUSY predicts that every Standard Model particle has a SUSY counterpart.'
Then there's the puzzle of antimatter: in the early Universe matter and antimatter were created in equal quantities but now matter dominates the Universe.
'We still do not know what caused the emergence of this asymmetry,' Daniela explains. 'We have finally discovered the Higgs boson: this special particle, a particle that does not carry any spin, might decay to dark matter particles and may even explain why the Universe is matter dominated.'
Discovering the Higgs boson was a huge achievement but now the race is on to understand it: a prospect that Daniela is particularly excited about.
'This particle is truly fascinating,' she says. 'Spin explains the behaviour of elementary particles: matter particles like the electron have spin 1/2 while force particles like the photon, which is responsible for the electromagnetic interaction, have spin 1. Spin 1/2 particles obey the Pauli principle that forbids electrons to be in the same quantum state.
'The Higgs is the first spin 0 particle, or as particle physicists would say the first 'scalar particle' we've found, so the Higgs is neither matter nor force.'
Because of its nature the Higgs could have an impact on cosmic inflation and the energy of a vacuum as well as explaining the mass of elementary particles.
Daniela tells me: 'Because of the Higgs the electron has mass, atoms can be formed, and we exist. But why do elementary particles have such difference masses? The data of run 2 will enable us to study, with higher precision, the decays of the Higgs boson and directly measure the coupling of the Higgs to quarks. It will also enable us to search for other particles similar to the Higgs and determine if the Higgs decays to dark matter.'
Daniela is one of 13 academics at Oxford working on ATLAS supported by a team of postdoctoral fellows, postgraduate students and engineering, technical, and computing teams. The Oxford group plays a lead role in operating the SemiConductor Tracker (SCT), most of which was assembled in an Oxford lab. This provides information on the trajectories of the particles produced when the LHC’s beams collide, which was crucial to the discovery of the Higgs boson.
Whilst the next few years will see the Oxford group busy with research that exploits the LHC’s new high-energy run, the team are also looking ahead to 2025 when the intensity or 'luminosity' of the beams will be increased.
The LHC is filled with 1,380 bunches of protons each containing almost a billion protons and colliding 40 million times per second. This means that every time two bunches of protons cross they generate not one collision but many, an effect called 'pile-up'.
'After this luminosity upgrade the LHC will operate at collision rates five to ten times higher than it does at present,' Daniela explains. 'In run 1 of the LHC we had a maximum of 37 pile-up collisions per crossing but with the upgrade to the High Luminosity LHC, or 'HL-LHC', this will increase to an average of 140 pile-up events in each bunch crossing.'
With the HL-LHC generating many more collisions, the international Oxford-led team are designing and prototyping parts of a new semiconductor tracker that will be needed to help reconstruct particles from the complex web of decay trails they leave inside the machine.
As the LHC ramps up both its energy and luminosity it promises to give scientists working on experiments such as ATLAS answers to some of the biggest questions in physics. One thing is certain: this new physics will also lead to a whole set of new questions about the matter that makes up us and the Universe around us.