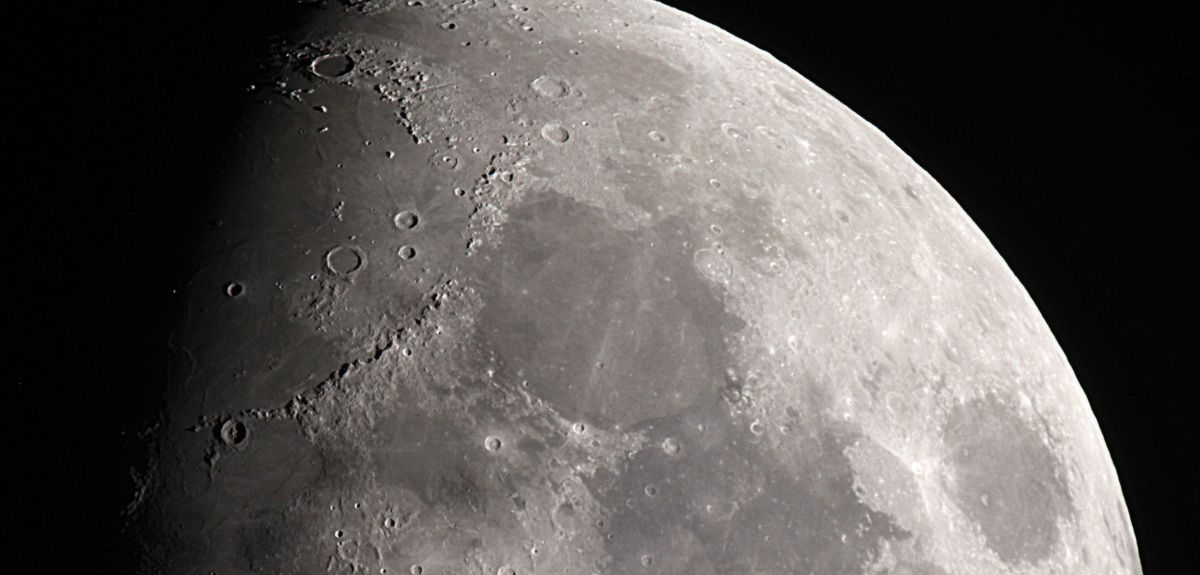
Professor Joe Silk on why we need to go back to the Moon
Professor Joe Silk. Image credit: Joe Silk.
He is currently an Emeritus Fellow of New College, a fellow of the American Academy of Arts and Sciences, the American Physical Society, the Institute of Physics, the Royal Society and the US National Academy of Sciences. Besides authoring more than 900 academic publications, he has written several popular books including ‘The Big Bang’, ‘The Infinite Cosmos’, ‘On the Shores of the Unknown: A Short History of the Universe’, and ‘Cosmic Enigmas.’
Despite having received multiple lifetime achievement awards, Professor Silk shows no sign of slowing down yet. His latest book ‘Back to the Moon: The Next Giant Leap for Humankind’ was published in November 2022. He talks about his career to date, and how returning to the Moon could help us uncover our cosmic origins.
In your latest book 'Back to the Moon' you argue that returning to the Moon could open up a ‘thrilling new age of scientific exploration.’ Please can you explain this?
The answer to that really goes back to the question that has driven my entire career: What is the underlying structure of the Universe and where did it come from?
We know that the Universe started as a relatively homogenous, smooth and dense distribution of mass that eventually developed into what we see today, with galaxies, stars, planets, and so on. Cosmologists such as myself are intensely interested in that evolutionary process, which we study using powerful telescopes. Quite literally, these enable us to see back in time – but not quite as far as we would like to. To see back before there were any galaxies, before there was any light, that’s our greatest challenge. We can only do that with radio waves that can probe the gas clouds of atomic hydrogen from which all galaxies assembled.
It’s essentially impossible from the Earth because we need to look in really low radio frequencies to see back this far. But on the far side of the Moon, conditions are unbelievably well optimised for probing the dark ages of the Universe, before the first galaxies formed. It is this epoch that holds clues to our cosmic beginnings.
What do we understand at the moment?
The furthest back we can see in time is the cosmic microwave background (CMB) radiation, dating from 400,000 years after the Big Bang, long before there were any galaxies or stars. Basically, the CMB is the cooled remnant of the first light to travel freely through the Universe, and we experience it as a radio signal that originates from every point in the sky. At first, this signal appeared to be completely uniform, but with highly precise telescopes we eventually detected small variations. These correspond to tiny fluctuations in temperature, caused by ‘ripples’ in the underlying structure. These ripples get stronger over time, until gravity eventually pulls them together to develop into gas clouds and then galaxies.
We are now beginning to capture this phenomenon using large microwave telescopes in space and in remote areas on Earth. We glimpse the seeds. But I want to study how these seeds assembled into galaxies. I need to go back in time to before there were any galaxies, to see their building blocks. To see the wisps of hydrogen gas from which galaxies assembled. To learn where these irregularities came from in the first place – to go right back to the ‘dark ages’ before there was any light at all.
If we could see further back in time, what could this tell us?
Our current best theory is that the Universe began as highly dense matter, then went through a dramatic period of inflation in a fraction of a second to reach its current proportions. From this remote origin, we believe that the ripples we see in the CMB today were created from tiny, high-energy quantum fluctuations that expanded during the end of the inflationary phase into cosmological proportions. If we could see back far enough to probe these fluctuations, this glimpse into the darkness could verify that inflation occurred during the first quadrillionth of a nano-second of the Universe’s existence.
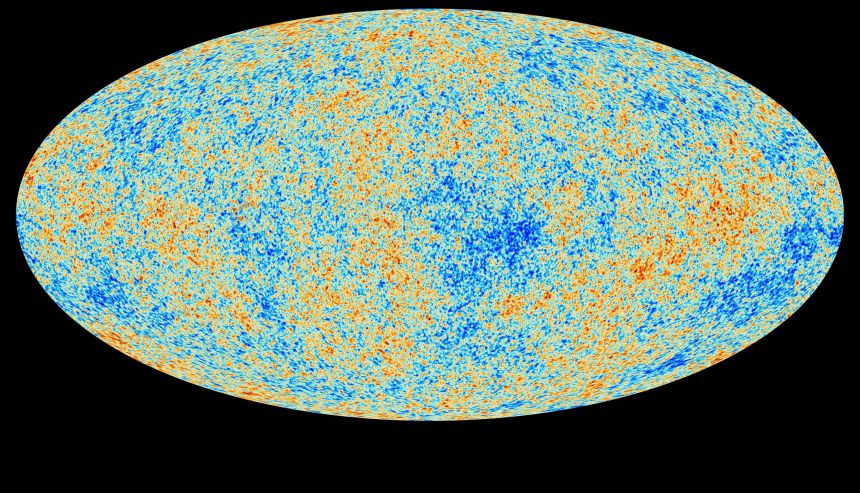
So, where does the Moon come into this?
We can’t see into the dark ages on Earth because a layer of ionized gas in our atmosphere acts as a surrounding blanket. The atmosphere is a good thing for life, but its ionosphere layer scatters low frequency radio wave signals. These are the ultimate messengers from the dark ages. In theory, the best place to set up a low frequency radio telescope would be in outer space, but this is extremely difficult for practical reasons. And even there we would be subject to radio interference from the Earth.
A more realistic prospect is the far side of the Moon. Because the Moon has no ionosphere, in terms of radio interference it is probably one of the quietest places in the inner solar system. And the far side of the Moon is shielded from all the radio waves coming from Earth, from mobile phone masts and maritime radar, for instance. It’s a stable platform where we could build incredibly large telescopes.
To be clear, this is a very long-term project that is only just beginning to undergo design studies. It could take anywhere between 10 and 30 years before these experiments come to fruition. But the journey is beginning, and even in the next few years we expect to be launching the first pathfinder projects to the far side of the Moon.
Besides mining, I envisage that the Moon will become a hub for lunar tourism and even interplanetary travel. Within its craters, there are huge resources of ice and water that could provide a source of hydrogen and oxygen for energy and rocket fuel. And because the Moon has a much lower gravity than Earth, it would make a great place to build a spaceport for rocket launches.
Are there other reasons we should go back to the Moon – for instance, to mine critical elements?
It is true that we are running out of semiconductor and rare earth materials that are critical for technologies such as computers and wind turbines. The Moon is a prime target for future mining expeditions because it is many thousands of times richer in these resources than Earth. This is due to its surface having been bombarded over billions of years by meteorites that have deposited layers of rare materials on its surface.
Besides mining, I envisage that the Moon will become a hub for lunar tourism and even interplanetary travel. Within its craters, there are huge resources of ice and water that could provide a source of hydrogen and oxygen for energy and rocket fuel. And because the Moon has a much lower gravity than Earth, it would make a great place to build a spaceport for rocket launches. The first step is likely to be NASA’s Artemis programme which envisages launches from a lunar space station, following the return of astronauts to the lunar surface within three or four years from now.
Apart from lunar exploration, what else are you researching at the moment?
What is the nature of the dark matter? That’s one of the greatest puzzles in astrophysics. Dark matter is the dominant form of matter in galaxies, and indeed in the Universe. Yet it is not the ordinary matter that stars are made of, and only interacts very weakly with ordinary matter. Most likely it consists of an as yet undiscovered elementary particle. I have proposed ingenious techniques for detecting such particles. For example, dark matter particles may interact with themselves. They can annihilate or decay, and produce electromagnetic signals that are potentially detectable, such as gamma rays.
There is a mysterious excess of gamma radiation from the centre of the Milky Way galaxy. That’s where the density of dark matter is highest. Perhaps this is a signal from dark matter, or perhaps it’s the cumulative emission from astrophysical sources such as a large abundance of rapidly spinning old neutron stars, which are known to be weak gamma ray sources. We don’t know and are seeking other tests, such as examining nearby dwarf galaxies, known repositories of dark matter. So far, the jury is out.
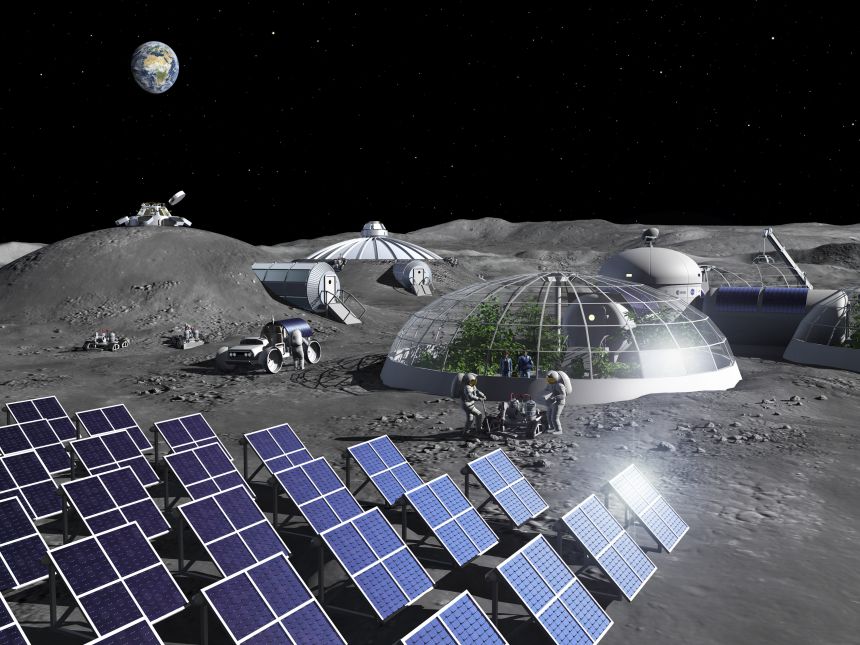
You were appointed as the University of Oxford’s Savilian Professor of Astronomy (from 1999 to September 2011), one of Oxford's oldest chair positions, founded in 1619. What were your key achievements in this role?
Oxford has a very illustrious past for astronomy, most notably the discovery around 1705 by Savilian Professor Edmond Halley in an attic observatory on New College Lane of the periodic appearances of the comet now named after him. But when I arrived some three and a half centuries later, the Savilian chair had somewhat lost its momentum in its impact on Oxford astronomy. I am proud of the role I played to drive cosmology forward, in particular by developing several major research themes in surveying distant galaxies, galaxy formation, and the nature of gravity.
During my period of leadership, I oversaw the appointments of several young researchers who have since evolved into international leaders in their various fields of research. I founded the Beecroft Institute for Particle Astrophysics and Cosmology in 2003, now a thriving hub of cosmology research. For instance, one of the leading numerical simulations of the Universe was developed at Oxford, and is now widely used to understand how the diverse properties of galaxies are generated. Another achievement was exploring alternatives to Einstein’s theory of gravity to monitor the relevance of challenges to our canonical model of structure formation, the cold dark matter theory, and to tensions in our measures of the rate at which the Universe is expanding.
I need to go back in time to before there were any galaxies, to see their building blocks. To see the wisps of hydrogen gas from which galaxies assembled. To learn where these irregularities came from in the first place – to go right back to the ‘dark ages’ before there was any light at all.
You have authored over 900 publications; which one are you most proud of?
This would have to be one of my first papers, ‘Fluctuations in the primordial fireball’, published in Nature in 1967. In this short paper, I predicted the presence of primordial temperature fluctuations in the CMB. I argued that tiny seeds, overdensities or underdensities in the intensity of radiation, had to be present at the beginning of time, as otherwise Einstein’s theory of gravity could not adequately explain galaxy formation. Detection would be a crucial test of gravitational theory and of the Big Bang itself. It took a very long time, around 30 years, but eventually these temperature fluctuations were found – one of the proudest moments of my life!
Have you always been interested in outer space?
Not at all! I grew up in London, so only saw the stars on occasional camping trips with the Boy Scouts. I actually read mathematics as an undergraduate at the University of Cambridge. During my final year, I found that the prospect of becoming an actuary or something like that left me rather bored. So, when I was procrastinating instead of revising for my final exams, I started wandering around lectures for different subjects, sitting at the back so no one would notice my ‘unauthorised’ presence.
I stumbled across the charismatic cosmologist Dennis Sciama, whose lectures blew my mind away. He was explaining how Albert Einstein was himself inspired by Mach’s principle. Ernst Mach was a 19th century physicist/philosopher who argued that the inertia of our local reference frame is determined by the distant stars – or to put it more simply, that local physical laws are determined by the large-scale distribution of matter in the Universe. I realised that our amazing Universe was motivating very deep questions that really fired me up.
This led me to do a diploma course in Physics at Manchester University. There I did a research project with an eminent radio astronomer, Roger Jennison, one of the founders of radio interferometry. This went so well that it opened the way to a graduate fellowship provided by the precursor of what is now the European Space Agency. I chose Harvard University because I wanted to work with cosmologist David Layzer, whose specialty was the formation of structure in the expanding universe. I was fortunate that I began my PhD at Harvard just after the major discovery of the CMB, which opened up a completely new and unexplored territory in cosmology that has sustained almost my entire career.
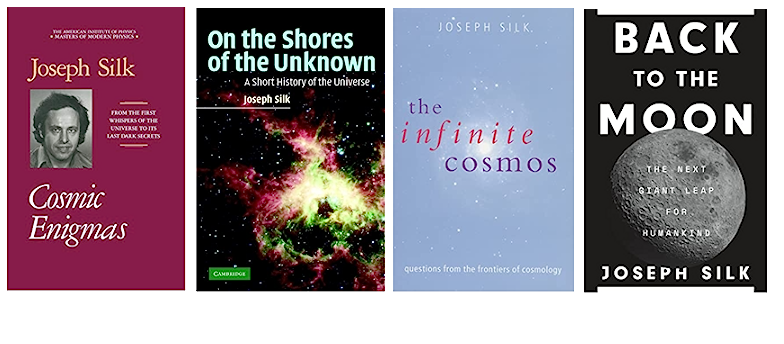
Do you think there is life out there?
That is the question that will always capture the public’s imagination. It is an exciting time now that we can probe the atmospheres of distant planets for signatures that could be indicative of life: oxygen, chlorophyll, methane, etc. But there really are no guarantees. It is hard to even estimate the number of planets we would need to look at to make discovering extra-terrestrial life a likely probability. Life is fragile, and it is likely to be a rare phenomenon. And the number of Earth-like planets that are relatively close to us is very limited.
There is only one way to pursue this goal. To find large numbers of Earth-like twins, that is planets with rocky cores, an atmosphere, and close to stars like our sun, we need a very large telescope in space. Realistically, that could best be constructed on the Moon – giving us another reason to invest in lunar exploration.
What have been some of the most exciting technological advancements in cosmology during your career?
Certainly, the incredible rise in computational power and our ability now to make very detailed, precise computer simulations of all sorts of phenomena. In my early career work on galaxy formation, my ‘models’ were cartoons along with a few simple equations. Now, using powerful computers, we can make incredible simulations of galaxies that are almost indistinguishable from the real thing.
You have written several cosmology books for the general public. How important is it that the public are interested in space science?
I do believe that it is important to engage the public with our work, and not just because they pay our bills and fund our research. The beauty and mystery of space has an ability to inspire awe that few things can come close to. So, it is only right that we open up our notebooks to the general public, and explain the rationale behind our goals in answering the great questions of the Universe. In this way, we build up interest and momentum to keep up what we are doing.
You can learn more about Professor Silk’s work in a series of video talks: The Physics of Fine-Tuning. These discuss how the laws of physics, the initial conditions of the universe, and features of our local world work together to produce the world we live in.
Glossary:
Dark matter: A proposed component of the Universe which cannot be seen because it does not absorb, reflect, or emit electromagnetic radiation. Its presence can only be inferred from its gravitational attraction on visible matter. Dark matter is thought to account for around 27% of the Universe; the rest is dark energy (68%) and normal visible matter (5%).