Features
Malaria kills more than 600,000 people every year, and each year, World Malaria Day (25th April) raises awareness of what continues to be one of the world’s deadliest infectious diseases.
The theme for this year’s World Malaria is on health equity across the globe, and the challenges faced by vulnerable groups such as pregnant women and young children.
Philippe Guerin was a clinician before working with Médecins Sans Frontières across Asia and Africa. He is now Professor of Epidemiology and Global Health at the University of Oxford, with a special interest in malaria. His work has provided evidence to support changes in the World Health Organization’s treatment guidelines for malaria.
'Malaria treatment guidelines are developed for the general population, but are rarely tailored for vulnerable subpopulations such as babies, pregnant women, and patients with comorbidities such as malnutrition, or HIV co-infections,' says Professor Guerin.
'It is imperative to develop optimised approaches for these groups too, to give them the best treatment for them. This is what is called precision public health.'
This is not because health authorities do not recognise the vulnerabilities these groups face - it is because there just wasn’t enough data to make clear recommendations. Vulnerable groups such as young children, pregnant women, or patients suffering from concomitant comorbidities are rarely included in clinical studies for a number of reasons, including ethical or safety concerns, costs, and recruitment challenges.
The problem is compounded by the fact that they are far fewer clinical studies of health conditions that affect the global south, compared to diseases that affect economically richer countries.
Figure 1: A graph comparing the number of published studies and clinical trials on different diseases. Diabetes, cancer, myocardial infarctions (heart attacks) are bigger concerns in the global north, while diseases such as malaria, schistosomiasis, chagas, Ebola, etc, affect populations almost exclusively in the global south.
Answering new research questions from existing data
'When it comes to diseases in resource limited settings, data are scarce and scattered: the number of scientific publications for something like diabetes is about 10 times that for malaria,' points out Professor Guerin.
One way to get around to this problem is to club together clinical data from individual patients into a larger, single dataset. Doing so can produce policy-changing scientific evidence from existing data, essentially allowing researchers to answer new scientific questions from old data.
The power of this approach was illustrated by a recent study led by Professor Guerin, which found that antimalarial treatments are more likely to fail in children with acute malnutrition.
'Malaria and malnutrition both affect poorer communities with limited research resources, so there just aren’t enough studies on the efficacy of antimalarial drugs in malnourished children, and past studies have contradictory results,' says Professor Philippe Guerin. 'So we used a different strategy to answer this question.'
The research team pooled individual patient data from 36 different antimalarial efficacy studies from 24 countries, that included body measurements (including height and weight) for the study participants. Using this information about weight versus height and age, the researchers could infer which children were likely to be malnourished (acute and chronic), and track outcomes specifically for this group.
'No one individual study included a large enough sample of malnourished children to uncover a clear relationship, but by combining information across many different studies, which each included few malnourished children, we were able to spot a clear pattern,' said Guerin.
'Clinical trial data have potentially bigger value than a single use'
This approach of combining data from many studies and then reusing it to answer new research questions, was pioneered by the WorldWide Antimalarial Resistance Network (WWARN), which brought together hundreds of scientists from more than three hundred institutes to pool their knowledge – and to identify gaps in this knowledge.
Established in 2009, Professor Guerin was WWARN’s first Director, and led a group of scientists attempting to bypass some of the unique challenges of malaria clinical research: 'Security concerns and pollical instability can often make it difficult or impossible to carry out a clinical trial in many countries affected by malaria. Limited resources in health systems can also make it difficult to enrol a representative sample of patients, as many patients aren’t able to access healthcare, making them invisible to clinicians,' says Professor Guerin.
'All of this means that clinical research in diseases of poverty often has relatively small sample sizes. This makes it harder to identify risk factors for patients, as these risk factors are relatively rare: if you’re only looking at a few patients, you might not have one of these rare patients, or you might not have enough of these patients to spot a pattern' says Professor Guerin. 'It was only be assembling lots of data together, under the aegis of WWARN, that we were able to understand what was happening in populations at risk for malaria.'
WWARN’s work identified that the standard dose of an otherwise effective antimalarial treatment, was actually too low for children under five for the combination dihydroartemsinin-piperaquine. This finding led the WHO to revise its official treatment guidelines.
Similarly, WWARN’s work on malaria in pregnancy has provided strong evidence to support WHO’s guidelines for malaria treatment for pregnant women, which were initially based on a much weaker evidence base.
This approach of clubbing together existing data also gets around the much more limited funding for studying diseases that largely affect people in the global south.
'Running a clinical trial can be 20 times more expensive if not more than clubbing together existing data and analysing it to answer the same research question,' says Professor Guerin. 'Clinical data here is scarce and precious – when the primary analysis is completed, there is an opportunity for maximising the added value of existing data.'
Equity in research
WWARN’s success showed that it was possible to produce policy-changing scientific evidence from existing data, and in 2016, the Infectious Diseases Data Observatory (IDDO) extended the same approach to other tropical infectious diseases.
Headed by Professor Philippe Guerin, IDDO’s main staff headquarters is in Oxford University’s Old Road Campus. IDDO promotes the reuse of individual patient data across the global infectious disease community, especially for malaria.
With over 15 people in the data management team alone, IDDO curates data that scientists across the world submit, harmonises them and connects them up with other datasets, and then makes the data available for free to any researcher, enabling scientists to answer new research questions from existing data.
It is particularly keen that research is led and informed by scientists and communities that are actually affected by the diseases: more than half of the IDDO curated data are used for individual patient data meta-analyses led by researchers from low and middle income countries, across Africa, Asia and Latin America.
IDDO also hosts TDR fellows, as part of a WHO career development programme which places researchers from disease-endemic countries with research organisations, to develop clinical research skills – IDDO will be welcoming two new TDR fellows this year. It also works with a number of institutions based in endemic regions including the Wellcome programmes in Asia and Africa, the EDCTP network of excellence (which brings together 15 European and 25 African countries), and the Indian Council of Medical Research to provide training.
'Tackling a disease like malaria needs many different kinds of expertise from many different places, and it is great to be working on a platform that brings many different kinds of scientists, from many different places, together', Professor Guerin says.
Find out more about IDDO’s resources to support malaria research on iddo.org/wwarn.
Professor Kamaldeep Bhui of Oxford's Department of Psychiatry, and former Editor-in-Chief of the British Journal of Psychiatry, explains the importance of editorial independence in promoting good science.
Given the contested nature of much mental health and brain research, it has never been more important for research in this area to be of the highest quality and observing the principles of research integrity and publication ethics. In so doing we ensure the production of knowledge does not bias the findings towards compounding health inequalities, with adverse policy or practice impacts.
As a psychiatrist and former editor of a scientific journal, I believe that editorial independence, through robust practice of publication ethics and research integrity, promotes good science and prevents bad science.
When editorial independence is challenged, we should all be concerned. This is why, together with senior members of the editorial team, I wrote an editorial for the British Journal of Psychiatry, the journal for which I used to be Editor-in-Chief.
Editorial independence in journals can be defined as editors having the freedom to make decisions about the scientific publication record. To promote good science, editorial independence must be non-negotiable for all scientific journals seeking to prevent influence from their owners or from groups with vested interests.
However, editorial independence is a multidimensional, dynamic, and complex concept that is often constructed in our responses to new and emerging challenges.
In the editorial, I outlined that too much published research is unsound with up to 10% of large-scale randomised trials suffering from major flaws. Corrections and retractions are one solution and should not be stigmatised. Rather they should be an accepted part of the contract between authors and editors, and a wider group of stakeholders. All should work in trust to establish and correct the scientific record on which public policy and practice can improve.
Dealing with older papers is especially complex. Scientific knowledge is contextual. Dismissing ‘old’ research entirely based on modern standards may overlook their incremental contributions. However, older papers should be appraised and judged against best practice at the time of publication; if found to be flawed in some fundamental scientific way, removal from the scientific record is appropriate.
Poor studies remain in the scientific record. There are hardly any requests for correction or retraction of uninfluential or uncited papers. Therefore, there is often a pressure to not retract a paper which is contributing to controversy and debate, as it rather perversely raises the profile of a journal. Indeed, many retracted or unretracted discredited papers continue to be highly cited.
Some larger publishers take charge of complaints, potential retractions, and any legal threats rather than following the guidance on editorial independence. This risks vested non-scientific interests becoming the basis or appearing to be the basis for decisions about flawed science.
An alarming trend is the use of legal threat to strategically control the publication and dissemination of public scientific findings. Legal challenges to editorial independence are especially difficult as settlements can be costly. To avoid this risk, owners may choose to neglect editorial decisions and undermine editorial independence. Our wider duties must also consider standards in public life and our respective professional values and codes of conduct.
When there are complaints or allegations of error, it is critical that the editor and author discuss potential remedies, for example corrections, re-analysis, the reporting and interpretation of the findings. This step should precede any discussion of potential retraction. When retraction is necessary, this should be mutually agreed if possible. A mutually satisfactory decision to retract may not be possible if authors object, or worse, if they resort to legal threat, thereby blocking any meaningful dialogue about the validity of their research.
As a former Editor in Chief and researcher myself, I know that the most important quality-control mechanism for research integrity is editorial independence guided by publication ethics to ensure that there is an uncompromising insistence on meeting the highest standards of scientific research, reporting and publication. Only then can improvements in practice and policy be grounded in science rather than vested interests.
Compliance with best practice guidance varies. Therefore, in our editorial, we suggested how we could protect and strengthen editorial independence. For example, regulation could include a register of breaches and scrutiny to learn lessons, as well as provision for legal advice on issues of public interest. Compliance with guidance, and adequate insurance and transparency must accompany the reconciliation of ethical and legal dilemmas. Scientists might disagree, the public and organisations representing specific positions might disagree; but the whole health industry from researcher, policy maker, to journal owners and editors must ensure they are capable and competent to fulfil obligations to protect editorial independence. Mapping policy and practice impacts of breaches, transparent processes for noting legal threats, and ethical leadership must sit alongside robust governance to support publication ethics and the principles and guidance on editorial independence.
Our early experiences can have a staggering impact on the rest of our lives – for better or worse. For young girls forced into marriages or unions against their will, usually with adult men, this can have profound lifelong consequences, including for wellbeing, education, and employment opportunities. Reducing early marriage can take many years of culture change, societal pressure, and policy change to transform the situation.
This is a strong example of how really robust, high-quality longitudinal research combining both quantitative and qualitative data can directly influence national policies to improve the lives of children and young people.
Dr Alan Sanchez, Young Lives Senior Quantitative Researcher
But along this journey, there are often turning points: pivotal moments that propel future progress.
In Peru, such a moment came on 25 November 2023 when the Government introduced new legislation to prohibit all marriages with minors under the age of 18. The potential impacts of this cannot be overstated: between 2013 and 2022, over 4,350 child marriages involving girls between the ages of 11 and 17 years were registered. Almost all (98%) of these resulted in young girls being married to adult men. But this is likely only the tip of the iceberg: estimates based on census data indicate that over 56,000 children aged between 12 and 17 (mostly girls) may be involved in a union and/or cohabitation.
This new law has closed a previous legal loophole which permitted teenagers to be married from the age of 14 years under certain conditions, with consent from at least one parent, despite the minimum legal age of marriage of 18 years for both girls and boys. Not only does the new law close this loophole, it also enables girls who were married as minors to have their marriages annulled.
On the surface, this may seem simply like a formal legislative process, but behind the scenes are years of evidence-gathering, lobbying, and policy engagement. In particular, the Young Lives study coordinated by the University of Oxford played a transformative role in building a compelling evidence base to champion the need for change.
Data that fills in the gaps
In combination, the Young Lives data fills in a key evidence gap by giving a personal glimpse into what early marriage is actually like in these countries. Beyond the high-level statistics, there are personal stories that resonate.
Julia Tilford, Communications Manager for Young Lives
‘It is rare that you can draw a direct link between academic research and policy change, but in this case, it is unequivocal’ says Julia Tilford, Communications Manager for Young Lives. Since 2001, the project has been following the lives of 12,000 young people in Ethiopia, India, Peru and Vietnam, using a combination of surveys (conducted every 3 to 4 years) and supplementary in-depth interviews to generate high-quality, large-scale longitudinal data on the causes and consequences of poverty and inequality. From 2005, Young Lives has been led by the Department of International Development at the University of Oxford. However, Young Lives also works in collaboration with key partners in each of the four study countries, who are engaged directly with national policy and programme decision-makers – those in a position to effect change. Young Lives has also worked with Child Frontiers to conduct in-depth interviews on the lived experience of early and child marriage and parenthood in Ethiopia, India, Peru and Zambia.
Understanding the long-term impacts of early marriage for children and young people in poor communities is one of many aspects the study has holistically examined. It has demonstrated that girls who marry early are less likely to complete secondary education, leading to reduced opportunities to gain employment and financial independence. Girls from poor households and rural areas typically have limited knowledge about or access to sexual and reproductive health care and services, increasing the risk of teenage pregnancies. Furthermore, children born to young mothers (under the age of 18) generally have a lower birthweight and shorter height for their age. Girls who marry early are also at much greater risk of physical and psychosocial violence from their partners.
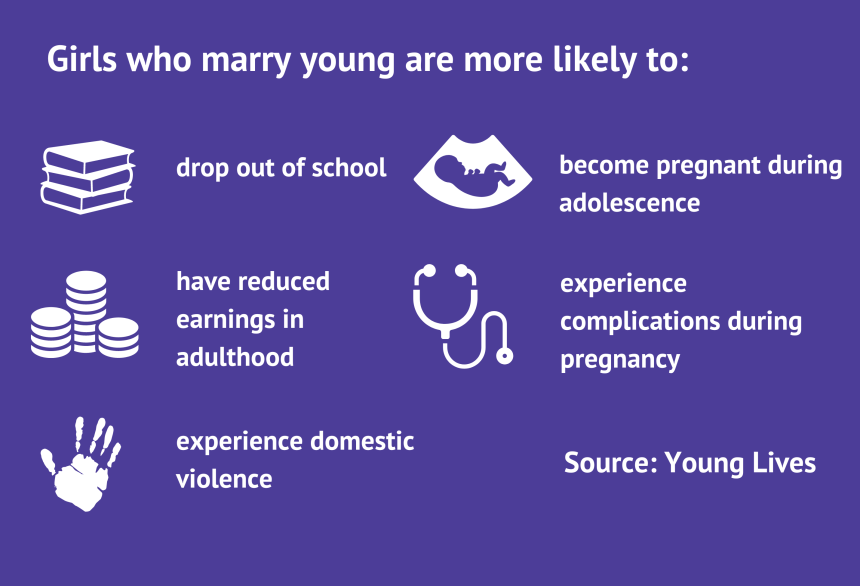
Every year, at least 12 million girls are married before they reach the age of 18. That is 28 girls every minute (UNICEF).
Translating research to policy impact
Leading up to the new Parliamentary Bill, Young Lives’ Peru team presented evidence on the impacts of early marriage and cohabitation through numerous presentations and briefings to key government officials, including in the Ministry of Women and Vulnerable Populations and the Ministry of Education. When the bill was presented to Congress in September 2022, Young Lives evidence was directly cited by Congresswoman Flor Pablo, who then invited Young Lives team members to present their findings at an influential congressional roundtable.
Being on the ground for over twenty years has enabled our Country Directors to establish long-term partnerships of trust and collaboration with key government partners and policy decision-makers. This enables Young Lives to be very effective at influencing national policies and programmes.
Kath Ford, Senior Policy Officer for Young Lives.
Dr Alan Sanchez, Young Lives Senior Quantitative Researcher and part of the group who presented at the roundtable, says: ‘Having such a robust evidence base was critical, because many people in Peru didn’t actually believe that early marriage had negative consequences.’
Alongside engaging with policy makers, Young Lives has also communicated its findings more widely – developing an animation, and working with the national press, which led to the findings being cited in high-profile articles in El Comercio and La República. The momentum built by these actions proved critical to keep the bill in progress when a political storm unleashed following the Peruvian President’s removal in December 2022 after an attempted coup d’état. Although the debate was postponed because of this, the bill eventually passed with 97% of Congress in favour.
‘What I have learnt from this process is that change takes time’ adds Dr Sanchez. ‘Besides the years needed to build up our strong longitudinal evidence base, it also takes time to analyse the data and build up support in Congress. You also need teamwork and political allies. In this case, having the support of Congresswoman Pablo was pivotal. She was personally motivated to change the law, and championed our evidence with key government ministers. In the end, even those who were political adversaries agreed that the bill was needed.’
Looking Forward
‘While the change in Peru’s legislation is an incredibly important step in protecting young girls from child marriage, legislation alone is not enough’ says Kath Ford, Senior Policy Officer for Young Lives. ‘The Young Lives study shows that poverty, gender inequality, and discrimination are key drivers of child marriage, meaning that policymakers need to adopt a broad approach to deliver real change for girls and young women. Tackling the root causes of child marriage also involves working closely with whole communities, including men and boys, and ensuring vulnerable girls and young women are protected by effective safety nets.’
Young Lives longitudinal evidence on the causes and consequences of child marriage in Peru has been pivotal for driving this important legislative change. By giving voice to the lived experiences of girls and young women, the study has enabled a much more in-depth understanding of how poverty and entrenched gender norms continue to drive child marriage, particularly among remote and indigenous communities.
Congresswoman Flor Pablo
Young Lives evidence on the consequences of early marriage is also starting to have a significant impact in India, where nearly 1 in 4 young women are married before the age of 18 (UNICEF, 2022). The Young Lives India team were invited to present evidence to the Indian Parliamentary Standing Committee as part of their examinations of a proposed new bill to increase the legal age of marriage for women from 18 to 21 years of age. This would be a seismic policy shift for a country where the majority of young women currently get married between the ages of 18 and 21.
Dr Renu Singh, Country Director, Young Lives India, says: ‘Young Lives has raised the importance of curbing child marriage and brought this important issue to the attention of policy makers over a number of years. The fact that the bill to raise the marriage of girls from 18 to 21 years has been passed by Cabinet, the first critical legislative step, before being ratified by Parliament is testament to this ongoing pressure.'
Meanwhile, the Young Lives team in Oxford are now completing the latest round of survey interviews. With the respondents now aged 22 and 29, the study is now focusing on how they are faring in young adulthood across a broad range of areas, including physical and mental health, education, work, and family relationships. New research findings will be published in early 2025.
You can learn more about Young Lives on the project's website.

Brian Christian is an acclaimed American author and researcher who explores the human and societal implications of computer science. His bestselling books include ‘The Most Human Human’ (2011), ‘Algorithms to Live By’ (2016), and ‘The Alignment Problem’ (2021), the latter of which The New York Times said ‘If you’re going to read one book on artificial intelligence, this is the one.’ He holds a degree from Brown University in computer science and philosophy and an MFA in poetry from the University of Washington.
Here, Brian talks about the latest chapter of his career journey: starting a DPhil (PhD) at the University of Oxford to grapple with the challenge of designing AI programs that truly align with human values.
Brian at the entrance of Lincoln College, Oxford. Credit: Rose Linke.
One of the fun things about being an author is that you get to have an existential crisis every time you finish a book. When The Alignment Problem was published in 2021, I found myself ‘unemployed’ again, wondering what my next project would be. But writing The Alignment Problem had left me with a sense of unfinished business. As with all my books, the process of researching it had been driven by a curiosity that doesn’t stop when it reaches the frontiers of knowledge: what began as scholarly and journalistic questions simply turned into research questions. I wanted be able to go deeper into some of those questions than a lay reader would necessarily be interested in.
The Alignment Problem is widely said to be one of the best books about artificial intelligence. How would you summarise the central idea of the book?
The alignment problem is essentially about how we get machines to behave in accordance with human norms and human values. We are moving from traditional software, where behaviour is manually and explicitly specified, to machine learning systems that essentially learn by examples. How can we be sure that they are learning the right things from the right examples, and that they will go on to actually behave in the way that we want and expect?
This is a problem which is getting increasingly urgent, as not only are these models becoming more and more capable, but they are also being more and more widely deployed throughout many different levels of society. The book charts the history of the field, explains its core ideas, and traces its many open problems through personal stories of about a hundred individual researchers.
Most AI systems are built on the assumption that humans are essentially rational but we all act on impulse and emotionally at times. However, there is currently no real way of accounting for this in the algorithms underpinning AI.
So, how does this relate to your DPhil project?
Under the supervision of Professor Chris Summerfield in the Human Information Processing lab (part of the Department of Experimental Psychology), I am exploring how cognitive science and computational neuroscience can help us to develop mathematical models that capture what humans actually value and care about. Ultimately, these could help enable AI systems that are more aligned with humans – and which could even give us a deeper understanding of ourselves.
For instance, most AI systems are built on the assumption that humans are essentially rational utility maximisers. But we know this isn’t the case, as demonstrated by, for instance, compulsive and addictive behaviours. We all act on impulse and emotionally at times - that’s why they put chocolate bars by the checkouts in supermarkets! But there is currently no real way of accounting for this in the algorithms underpinning AI.
To me, this feels as though the computer sciences community wrote a giant IOU: ‘insert actual model of human decision making here.’ There is a deep philosophical tension between this outrageous simplification of human values and decision making, and their use in systems that are becoming increasingly powerful and pervasive in our societies.
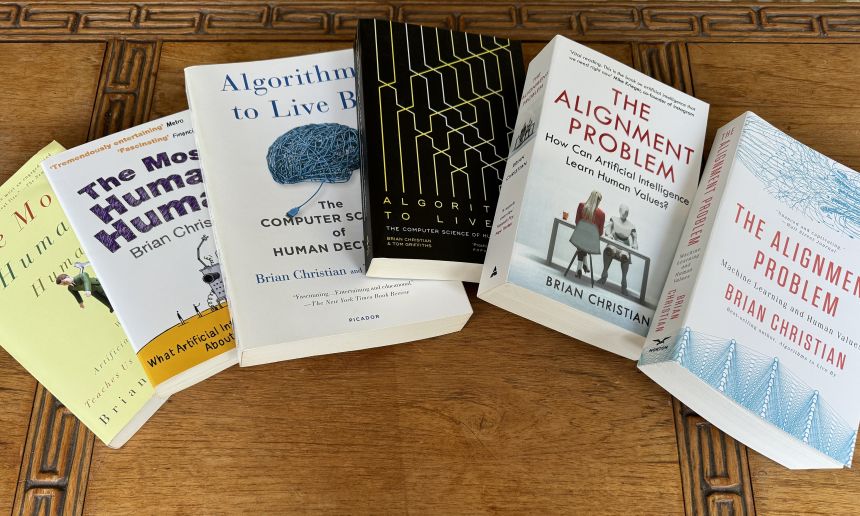
And how will you investigate this?
I am currently developing a roadmap with Chris and my co-supervisor Associate Professor Jakob Foerster (Department of Engineering Science) that will involve both simulations and human studies. One fundamental assumption in the AI literature that we are probing is the concept of “reward.” Standard reinforcement-learning models assume that things in the world simply have reward value, and that humans act in order to obtain those rewards. But we know that the human experience is characterized, at a very fundamental level, by assigning value to things.
There’s a famous fable from Aesop of a fox who, failing to jump high enough to reach some grapes, declares them to have been “sour” anyway. The fox, in other words, has changed their value. This story, which has been passed down through human culture for more than two millennia, speaks to something deeply human, and it offers us a clue that the standard model of reward isn’t correct.
This opens up some intriguing questions. For instance, what is the interaction between our voluntary adoption of a goal, and our involuntary sense of what the reward of achieving that goal will be? How do humans revise the value, not only of their goal itself but of the other alternatives, to facilitate the plan they have decided to pursue? This is one example of using the tools of psychology and computational neuroscience to challenge some of the longstanding mathematical assumptions about human behaviour that exist in the AI literature.
We are moving from traditional software, where behaviour is explicitly specified, to machine learning systems that essentially learn by examples. How can we be sure that they are learning the right things from the right examples, and that they will go on to actually behave in the way that we want?
Does the future of AI frighten you?
Over the years I have been frightened to different extents by different aspects of AI: safety, ethics, economic impact. Part of me is concerned that AI might systematically empower people, but in the wrong way, which can be a form of harm. Even if we make AI more aligned, it may only be aligned asymmetrically, to one part of the self. For instance, American TikTok users alone spend roughly 5 billion minutes on TikTok a day: this is equivalent to almost two hundred waking human lifetimes. A day! We have empowered, at societal scale, the impulsive self to get what it wants – a constant stream of diverting content – but there is more to a life well lived than that.
Do you feel academia has an important role in shaping the future of AI?
Definitely. What is striking to me is how academia kickstarted a lot of the current ideas behind AI safety and AI alignment – these started off on the whiteboards of academic institutions like Oxford, UC Berkeley, and Stanford. Over the last four years, those ideas have been taken up by industry, and AI alignment has gone from being a theoretical to an applied discipline. I think it is very important to have a heterogenous and diverse set of incentives, timescales, and institutional structures to pursue something as big, critical. and multidimensional as AI alignment.
Academia can also have more longevity than industrial research labs, which are typically at the mercy of profit motivations. Academia isn’t so tied to boom and bust cycles of funding. It might not be able to raise billions of dollars overnight, but it isn’t going to go away with the stroke of a pen or a shareholder meeting.
Oxford's unique college system really fosters a sense of community and intellectual exchange that I find incredibly enriching. It is inspiring, it gives you new ideas, and provides a constant reminder of how many open frontiers there are, how many important and urgent open problems.
Why did you choose Oxford for your PhD?
I came to Oxford many times to interview researchers while working on The Alignment Problem. At the time, the fields of AI ethics, AI safety, and AI policy were just being born, with a lot of the key ideas germinating in Oxford. I soon became enamoured of Oxford as a university, a town, and an intellectual community - I felt a sense of belonging and connection. So, Oxford was the obvious choice for where I wanted to do a PhD, and I didn’t actually apply to anywhere else! What sealed it was finding Chris Summerfield. He has a really unique profile as an academic computational neuroscientist who has worked at one of the largest AI research labs, Google DeepMind, and now at the UK’s AI Safety Institute. We met for coffee in 2022 and it all went from there.
What else do you like about being a student again?
Oxford's unique college system really fosters a sense of community and intellectual exchange that I find incredibly enriching. In the US, building a community outside your particular discipline is not really part of the graduate student experience, so I have really savoured this. It is inspiring, it gives you new ideas, and provides a constant reminder of how many open frontiers there are, how many important and urgent open problems. When you work in AI, there can be a tendency sometimes to think that it is the only critical thing to be working on, but this is obviously so far from the truth.
Outside of my work life, I play the drums, and a medium-term project is to find a band to play in. Amongst many other things, Oxford really punches above its weight on the music scene, so I am looking forward to getting more involved in that. Once the weather improves, I also hope to take up one of my favourite pastimes – going on long walks – to explore the wealth of surrounding landscape. As someone who spends a lot of time sitting in front of computers while thinking about computers, it is immensely refreshing to get away from the world of screens and step into nature.
The world’s most powerful computer hasn’t yet been built – but we have the blueprint, says the team behind Oxford spinout Quantum Dynamics.
Meanwhile, Chris Ballance, co-founder of spinout Oxford Ionics, says ‘quantum computing is already solving complex computing test cases in seconds – solutions that would otherwise take thousands of years to find.’
Following decades of pioneering research and development – much of it carried out at the University of Oxford –some of the world’s most innovative companies have integrated their technology into existing systems with excellent results.
Ask a quantum scientist at the University of Oxford for a helpful analogy and they may direct you towards the Bodleian library: a classical computer would look through each book in turn to find the hidden golden ticket, potentially taking thousands of years; an advanced quantum computer could simply open every book at once.
From drug discovery and climate prediction to ultra-powerful AI models and next-generation cryptology and cybersecurity, the potential applications of quantum computing are near-limitless. We’re not there yet, but a host of quantum-based companies with roots in Oxford academia are driving us towards a world of viable, scalable and functional quantum computers – and making sure we’ll be safe when we get there.
With the UK government’s recent announcement to inject £45 million into funding quantum computing research, the future is looking brighter for getting these technologies out into the real world.
Let’s meet some Oxford spinouts leading the charge.
Quantum Motion Technologies
Research suggests that, as with today’s smartphones and computers, silicon is the ideal material from which to make qubits – the basic units of quantum information and the building blocks of quantum computers. Formed in 2017 by Professor Simon Benjamin of Oxford’s Department of Materials and Professor John Morton at UCL, Quantum Motion is developing scalable architecture to take us beyond the current small, error-prone quantum computers.
PQShield
Any news article about the benefits of quantum computing is also likely to highlight a threat: the potential of quantum technology to shatter today’s encryption techniques (imagine a computer able to guess all possible password combinations at once). PQShield, spun out of Oxford’s Mathematical Institute by Dr Ali El Kaafarani, uses sophisticated maths to develop secure, world-leading ‘post-quantum’ cryptosystems. The team has the largest assembly of post-quantum crypto specialists in the world, servicing the whole supply chain.
Orca Computing
Making quantum computing a practical reality is what drives the team at Orca, spun out of research developed at the University of Oxford in 2019. The company is developing scalable quantum architecture using photonics – the manipulation of light – as its basis. In the short-term, that means creating usable technology derived from repurposing telecoms for quantum. This enables the team to build massive-scale information densities without resorting to impossible numbers of components. In the longer-term, Orca’s approach means error-corrected quantum computers with truly transformative potential. Dr Richard Murray, CEO and co-founder, explains: ‘Thanks to our drive towards delivering commercially realistic solutions, we are addressing the consumption of quantum.’
Oxford Ionics
Co-founded in 2019 by Dr Chris Ballance of Oxford’s Department of Physics, Oxford Ionics’ qubits are composed of individual atoms – the universe’s closest approximation to a perfect quantum system. These high-performance qubits have won Oxford Ionics a £6m contract to supply a quantum computer to the UK’s National Quantum Computing Centre in Harwell, Oxfordshire, with the aim of developing new applications.
QuantrolOx
To build practical quantum computers, scientists will need millions of physical qubits working in constant harmony – a big challenge to scaling up. QuantrolOx’s AI software automates the ‘tuning’ process, allowing quicker feedback and better performance. The company, co-founded by Professor Andrew Briggs of Oxford’s Department of Materials, envisions a world where every quantum computer will be fully automated.
Oxford Quantum Circuits
Oxford Quantum Circuits’ quantum computer is the only one of its kind commercially available in the UK. The company, founded by Dr Peter Leek of Oxford’s Department of Physics and today led by founding CEO Ilana Wisby, is driving quantum technology out of the lab and directly to customers’ fingertips, enabling breakthroughs in areas such as predictive medicine, climate change and AI algorithms. Ilana’s vision is for ten machines in ten countries within ten years..
Other innovations in processing power
As quantum computing continues to progress – but with the timeline for adoption unclear – innovators in Oxford are also looking for ways to turbocharge today’s computing technology.
Salience Labs
The speed of AI computation doubles every few months, outpacing standard semiconductor technologies. Salience Labs, a joint spinout of Oxford and Münster universities, is building photon-based – rather than electron-based – solutions to allow us to keep up with exponential AI innovation and the vast amounts of information that require processing in the 21st century.
Lumai
AI’s ability to analyse vast datasets at rapid pace is one of its big selling points. Trained models can produce diagnoses from a patient’s medical images or help insurance companies detect fraud. Oxford spinout Lumai works at the nexus of 3D optics and machine learning to provide an energy-efficient AI processor that delivers computation speeds 1,000 times faster than traditional electronics, enabling AI inference to move to the next level.
Machine Discovery
Harnessing its proprietary neural network technology, Machine Discovery makes complex numerical simulations quicker and cheaper. The Oxford Department of Physics spinout aims to provide its customers with all the benefits of machine learning – without the years of AI research. Its ‘Discovery Platform’ technology allows users to describe their problem and let the software find the solution.
- 1 of 247
- next ›